Chapter 17 Temperature, Heat & the First Law of Thermodynamics
Learning Objectives:
In this chapter you will basically learn:
\(\bullet\) Explain the zeroth law of thermodynamics.
\(\bullet\) Explain the conditions for the triple-point temperature.
\(\bullet\) Explain the conditions for measuring a temperature with a constant-volume gas thermometer.
\(\bullet\) Convert a temperature between any two (linear) temperature scales, including the Celsius, Fahrenheit, and Kelvin scales.
\(\bullet\) Identify that a change of one degree is the same on the Celsius and Kelvin scales.
\(\bullet\) For one-dimensional thermal expansion, apply the relationship between the temperature change %T, the length change \(\Delta L\), the initial length L, and the coefficient of linear expansion \(\alpha\).
\(\bullet\) For two-dimensional thermal expansion, use onedimensional thermal expansion to find the change in area.
\(\bullet\) For three-dimensional thermal expansion, apply the relationship between the temperature change %T, the volume change \(\Delta V\), the initial volume V, and the coefficient of volume expansion \(\beta\).
\(\bullet\) Identify that thermal energy is associated with the random motions of the microscopic bodies in an object.
\(\bullet\) Identify that heat Q is the amount of transferred energy (either to or from an object’s thermal energy) due to a temperature difference between the object and its environment.
\(\bullet\) Convert between mechanical or electrical energy and thermal energy.
\(\bullet\) For a temperature change \(\Delta T\) of a substance, relate the change to the heat transfer Q and the substance’s heat capacity C.
\(\bullet\) For a temperature change %T of a substance, relate the change to the heat transfer Q and the substance’s specific heat c and mass m.
\(\bullet\) Identify the three phases of matter.
\(\bullet\) For a phase change of a substance, relate the heat transfer Q, the heat of transformation L, and the amount of mass m transformed.
\(\bullet\) Identify that if a heat transfer Q takes a substance across a phase-change temperature, the transfer must be calculated in steps: (a) a temperature change to reach the phase-change temperature, (b) the phase change, and then (c) any temperature change that moves the substance away from the phase-change temperature.
\(\bullet\) If an enclosed gas expands or contracts, calculate the work W done by the gas by integrating the gas pressure with respect to the volume of the enclosure.
18.24 Apply the first law of thermodynamics to relate thecchange in the internal energy \(\Delta E_{int}\) of a gas, the energy Q transferred as heat to or from the gas, and the work W done on or by the gas.
\(\bullet\) Identify that the internal energy %Eint of a gas tends to increase if the heat transfer is to the gas, and it tends to decrease if the gas does work on its environment.
\(\bullet\) Identify that in an adiabatic process with a gas, there is no heat transfer Q with the environment.
\(\bullet\) Identify that in a constant-volume process with a gas, there is no work W done by the gas.
\(\bullet\) Identify that in a cyclical process with a gas, there is no net change in the internal energy \(\Delta E_{int}\).
\(\bullet\) Identify that in a free expansion with a gas, the heat transfer Q, work done W, and change in internal energy \(\Delta E_{int}\) are each zero.
\(\bullet\) For thermal conduction through a layer, apply the relationship between the energy-transfer rate Pcond and the layer’s area A, thermal conductivity k, thickness L, and temperature difference \(\Delta T\) (between its two sides).
\(\bullet\) For a composite slab (two or more layers) that has reached the steady state in which temperatures are no longer changing, identify that (by the conservation of energy) the rates of thermal conduction Pcond through the layers must be equal.
\(\bullet\) For thermal conduction through a layer, apply the relationship between thermal resistance R, thickness L, and thermal conductivity k.
\(\bullet\) Identify that thermal energy can be transferred by convection, in which a warmer fluid (gas or liquid) tends to rise in a cooler fluid.
\(\bullet\) In the emission of thermal radiation by an object, apply the relationship between the energy-transfer rate \(P_{rad}\) and the object’s surface area A, emissivity \(\varepsilon\), and surface temperature T (in kelvins).
\(\bullet\) In the absorption of thermal radiation by an object, apply the relationship between the energy-transfer rate \(P_{abs}\) and the object’s surface area A and emissivity \(\varepsilon\), and the environmental temperature T (in kelvins).
\(\bullet\) Calculate the net energy-transfer rate \(P_{net}\) of an object emitting radiation to its environment and absorbing radiation from that environment.
17.1 TEMPERATURE
Temperature
Temperature is one of the seven SI base units as given in Table 1-1. Our laboratory measurements of temperature in Physics are on the Kelvin scale, which is marked in units called kelvins. We are near a huge heat source or a star, a shining Sun that keeps us providing heat with an optimal amount. After Big Bang happened about 13.77 billion years ago the universe was very hot and its temperature was about \(10^{39} K\). Now the average temperature of the universe is about 3 K. However, we are living in a relatively higher temperature on Earth due to Sun at an average distance of about 150 million kilometers. According to measurements made by the most recent NASA satellite missions solar radiation we are receiving on the upper atmosphere directly facing the Sun is about 1,360 watts per square meter. The existence of humans, animal kingdom and other living creatures on Earth due to the heat we are receiving from the Sun and keeping us much warmer than the average temperature of 3 K of the universe. Although the temperature of a body apparently has no upper limit, it does have a lower limit; this limiting low temperature is taken as the zero of the Kelvin temperature scale. Room temperature is about 290 kelvins, or 290 K as we write it, above this absolute zero.
Figure 17-1 shows a wide range of temperatures on various references.
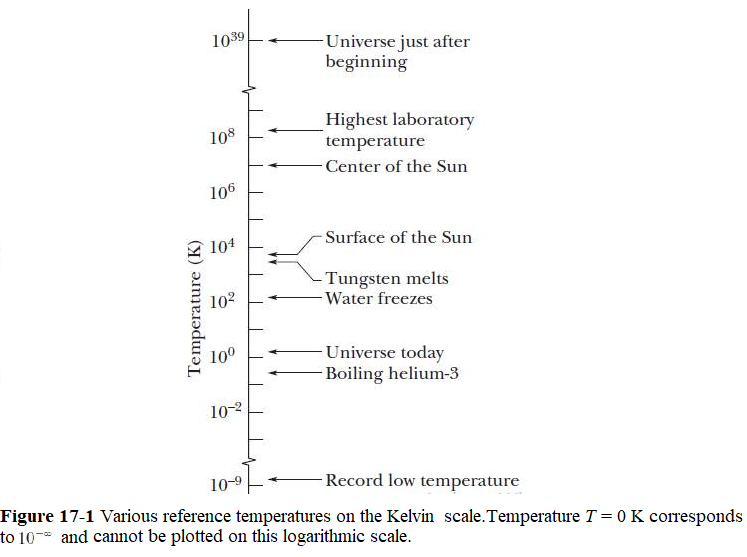
The Zeroth Law of Thermodynamics
If bodies A and B are each in thermal equilibrium with a third body T, then A and B are in thermal equilibrium with each other.
Measuring Temperature
The Triple of Water
The Constant-Volume Gas Thermometer
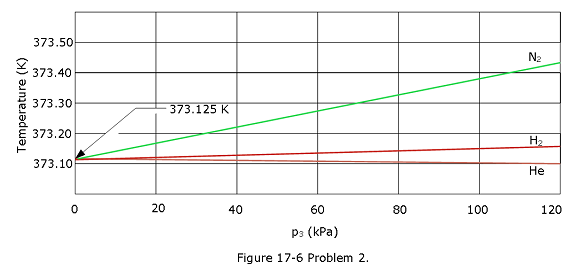
17.2 THE CELSIUS AND FAHRENHEIT SCALES
Table 17-1 Some Corresponding Temperatures
Temperature | \(^\circ C\) | \(^\circ F\) |
---|---|---|
Boiling point of water\(^a\) | 100 | 212 |
Normal body temperature | 37.0 | 98.6 |
Accepted comfort level | 20 | 68 |
Frezing point of water\(^a\) | 0 | 32 |
Zero of Farenheit scale | \(\approx -18\) | 0 |
Scales coincide | -40 | -40 |
17.3 THERMAL EXPANSION
Table 17-2 Some Coefficients of Linear Expansion\(^a\)
Substance | \(\alpha(10^{-6}/C^\circ)\) |
---|---|
Ice at \(0^\circ C\) | 51 |
Lead | 29 |
Aluminum | 23 |
Brass | 19 |
Copper | 17 |
Concrete | 12 |
Steel | 11 |
Ordinary glass | 9 |
Pyrex glass | 3.2 |
Diamond | 1.2 |
Invar\(^b\) | 0.7 |
Fused quartz | 0.5 |
17.4 ABSORPTION OF HEAT
Table 17-3 Some Specific Heats and Molar Specific Heats at Room Temperature
Specific Heat | Specific Heat | Molar Heat | |
---|---|---|---|
\(\frac{cal}{g.K}\) | \(\frac{J}{kg.K}\) | \(\frac{J}{mol.K}\) | |
Elemental Solids | |||
Lead | 0.0305 | 128 | 26.5 |
Tungsten | 0.0321 | 134 | 24.8 |
Silver | 0.0564 | 236 | 25.5 |
Copper | 0.0923 | 386 | 24.5 |
Aluminum | 0.215 | 900 | 24.4 |
Other Solids | |||
Brass | 0.092 | 380 | |
Granite | 0.19 | 790 | |
Glass | 0.20 | 840 | |
Ice($10-C) | 0.530 | 2220 | |
Liquids | |||
Mercury | 0.033 | 140 | |
Ethyl Alcohol | 0.58 | 2430 | |
Seawater | 0.93 | 3900 | |
Water | 1.00 | 4187 |
Table 18-4 Some Heats of Transformation
Melting | Boiling | |||
---|---|---|---|---|
Substance | Melting Point (K) | Heat of Fusion \(L_F\) (kJ/kg) | Boiling Point (K) | Heat of Fusion \(L_F\) (kJ/kg) |
Hydrogen | 14.0 | 58.0 | 20.3 | 455 |
Oxygen | 54.8 | 13.9 | 90.2 | 213 |
Mercury | 234 | 11.4 | 630 | 296 |
Water | 273 | 333 | 373 | 2256 |
Lead | 601 | 23.2 | 2017 | 858 |
Silver | 1235 | 105 | 2323 | 2336 |
Copper | 1356 | 207 | 2868 | 4730 |
17.5 THE FIRST LAW OF THERMODYNAMICS
Table 18-5 The First Law of Thermodynamics: Four Special Cases
The Law: \(\Delta E_{int}\) = Q - W | ||
---|---|---|
Process | Restriction | Consequence |
Adiabatic | Q = 0 | \(\Delta E_{int}=-W\) |
Constant Volume | W=0 | \(\Delta E_{int}=Q\) | Closed Cycle | \(\Delta E_{int}=0\) | Q=W |
Free Expansion | Q=W=0 | \(\Delta E_{int}=0\) |
17.6 HEAT TRANSFER MECHANISMS
Table 17-6 Some Thermal Conductivities
Substance | \(k(\frac{W}{m.K})\) |
---|---|
Metals | |
Stainless steel | 14 |
Lead | 35 |
Iron | 67 |
Brass | 109 |
Aluminum | 235 |
Copper | 401 |
Silver | 428 |
Gases | |
Air (dry) | 0.026 |
Helium | 0.15 |
Hydrogen | 0.18 |
Building Materials | |
Polyurethane foam | 0.024 |
Rock wool | 0.043 |
Fiberglass | 0.048 |
White pine | 0.11 |
Window glass | 1.0 |
Solved Problems:
- [3] A gas thermometer is constructed of two gas-containing bulbs, each in a water bath, as shown in Fig. 17-30.The pressure difference between the two bulbs is measured by a mercury manometer as shown. Appropriate reservoirs, not shown in the diagram, maintain constant gas volume in the two bulbs.There is no difference in pressure when both baths are at the triple point of water. The pressure difference is 120 torr when one bath is at the triple point and the other is at the boiling point of water. It is 90.0 torr when one bath is at the triple point and the other is at an unknown temperature to be measured.What is the unknown temperature?
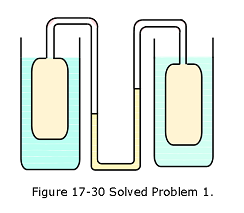
Solution:
\[T_{bp}-T_{tp}=(273.16\space K)\frac{p_{bp}-p_{tp}}{p_3}\] \[373.125\space K-273.16\space K=(273.16\space K)\frac{120\space Torr}{p_3}\] \[p_3=327.91\space Torr\] \[T_{un}-T_{tp}=(273.16\space K)\frac{p_{tp}-p_{un}}{p_3}\] \[T_{un}=T_{tp}+(273.16\space K)\frac{p_{tp}-p_{un}}{p_3}\] \[T_{un}=273.16\space K+(273.16\space K)\frac{90\space Torr}{327.91\space Torr}=348.13\space K\space(Answer)\] 2. [5] At what temperature is the Fahrenheit scale reading equal to (a) twice that of the Celsius scale and (b) half that of the Celsius scale?
Solution:
- \[T_F = \frac{9}{5}T_C+32^\circ\] \[2T_C - \frac{9}{5}T_C=32^\circ\] \[T_C = 160^\circ\space C\space(Answer)\]
- \[\frac{T_C}{2} - \frac{9}{5}T_C=32^\circ\] \[T_C = -24.62^\circ\space C\space(Answer)\]
- [21] As a result of a temperature rise of 32 \(C^\circ\), a bar with a crack at its center buckles upward (Fig. 17-32). The fixed distance \(L_0\) is 3.77 m and the coefficient of linear expansion of the bar is \(25 \times 10^{-6}/C^\circ\). Find the rise x of the center.
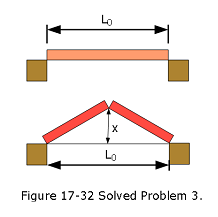
Solution: \[\Delta L = L_0\alpha \Delta T\] \[L-L_0 = L_0\alpha \Delta T\] \[L=L_0 + L_0\alpha \Delta T=L_0(1+\alpha\Delta T)=3.77\space m(1+25\times 10^{-6}/^\circ C\times32^\circ C)\] \[L=3.773016\space m\] \[\left(\frac{L}{2}\right)^2=x^2+\left(\frac{L_0}{2}\right)^2\] \[x=\sqrt{\left(\frac{L}{2}\right)^2-\left(\frac{L_0}{2}\right)^2}\] \[x=\sqrt{\left(\frac{3.773016\space m}{2}\right)^2-\left(\frac{3.77\space m}{2}\right)^2}=7.533\times 10^{-2}\space m\space(Answer)\] 4. [42] A 20.0 g copper ring at \(0.000^\circ\)C has an inner diameter of D = 2.54000 cm. An aluminum sphere at 100.0 \(^\circ\)C has a diameter of d = 2.545 08 cm. The sphere is put on top of the ring (Fig. 17-36), and the two are allowed to come to thermal equilibrium, with no heat lost to the surroundings. The sphere just passes through the ring at the equilibrium temperature. What is the mass of the sphere?
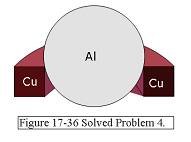
Solution:
Given at \(T=0.000\space ^\circ C\) \[m_{cu}=20\space g=0.020\space kg\] \[D_{cu}=2.54000\space cm=0.0254000\space m\] and given at \(T=100\space ^\circ C\) \[d_{al}=2.54508\space cm=0.0254508\space m\] Heat lost by aluminum + heat gained by copper=0 \[Q_{al}+Q_{cu}=0\] \[Q_{al}=-Q_{cu}\] \[c_{al}m_{al}(T_f-100^\circ C)=-c_{cu}m_{cu}(T_f-0^\circ C)\] \[m_{al}=-\frac{c_{cu}m_{cu}T_f}{c_{al}(T_f-100^\circ C)}\] But we need \(T_f\) after aluminum sphere and copper ring come to an equilibrium. At equilibrium d = D.
\[d_0+d_0\alpha_{al}(T_f-100)=D_0+D_0\alpha_{cu}(T_f-0)\] \[T_f=\frac{d_0(\alpha_{al}100-1)+D_0}{d_0\alpha_{al}-D_0\alpha_{cu}}\] \[T_f=\frac{2.5408~cm(23\times 10^{-6}/^\circ C\times 100-1)+2.54000~cm}{2.5408~cm \times23\times 10^{-6}/^\circ C-2.54000~cm \times17\times 10^{-6}/^\circ C}=50.38\space ^\circ C\] \[m_{al}=-\frac{c_{cu}m_{cu}T_f}{c_{al}(T_f-100^\circ C)}=-\frac{386\frac{J}{kg.K} (0.020~kg)(50.38^\circ C)}{900\frac{J}{kg.K}(50.38-100)^\circ C}=0.008709\space kg\space(Answer)\]
- [43] In Fig. 17-37, a gas sample expands from \(V_0\) to \(4.0V_0\) while its pressure decreases from \(p_0\) to \(p_0/4.0\). If \(V_0 = 1.0 ~m^3\) and \(p_0\) = 40 Pa, how much work is done by the gas if its pressure changes with volume via (a) path A, (b) path B, and (c) path C?
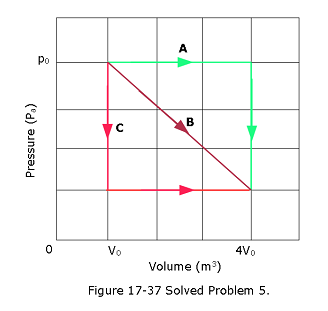
Solution:
Given \(V_0=1.0\space m^3\) and \(p_0=40\space Pa\)
\[W_A=p_0\Delta V = (40\space Pa)(4V_0-V_0)= 120\space J\space(Answer)\]
\[W_B=\frac{1}{2}(4V_0-V_0)(40-10)P_a+(4V_0-V_0)10=(45+30)\space J= 75\space J\space(Answer)\]
Work done by path C \[W_C= (4V_0-V_0)(10\space P_a)=30\space J\space (Answer)\]
- [60] Figure 17-46 shows the cross section of a wall made of three layers. The layer thicknesses are \(L_1\), \(L_2\) = 0.700\(L_1\), and \(L_3\) = 0.350\(L_1\). The thermal conductivities are \(k_1\), \(k_2\) = 0.900\(k_1\), and \(k_3\) = 0.800\(k_1\). The temperatures at the left side and right side of the wall are \(T_H\) = 30.0\(^\circ\)C and TC = -15.0 \(^\circ\)C, respectively. Thermal conduction is steady. (a) What is the temperature difference \(\Delta T_2\) across layer 2 (between the left and right sides of the layer)? If \(k_2\) were, instead, equal to \(1.1k_1\), (b) would the rate at which energy is conducted through the wall be greater than, less than, or the same as previously, and (c) what would be the value of \(\Delta T2\)?
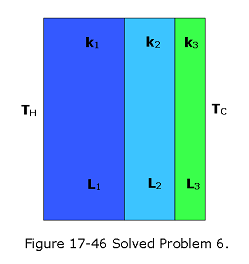
Solution:
Given \(L_2=0.700L_1\),\(L_3=0.350L_1\), \(k_2=0.900k_1\), \(k_3=0.8000k_1\),\(T_H=30^\circ C\) and \(T_C=-15.0^\circ C\).
- What is the temperature difference \(\Delta T_2\) across layer 2? The rate \(P_{cond}\) at which energy is conducted through a slab for which one face is maintained at the higher temperature \(T_H\) and the other face is maintained at the lower temperature \(T_C\) is
\[P=\frac{Q}{t}=kA\frac{T_H-T_C}{L}=A\frac{T_H-T_C}{\sum\frac{L}{k}}\] Here each face of the slab has area A, the length of the slab (the distance between the faces) is L, and k is the thermal conductivity of the material.
Using the above equation, we get \[P_{cond}=\frac{A(T_H-T_C)}{\sum\frac{L}{k}}=\frac{A(T_H-T_C)}{\frac{L_1}{k_1}+\frac{L_2}{k_2}+\frac{L_3}{k_3}}=\frac{A(T_H-T_C)}{\frac{L_1}{k_1}+\frac{0.700L_1}{0.900k_1}+\frac{0.350L_1}{0.800k_1}}=\frac{144Ak_1(T_H-T_C)}{319L_1}~~~(1)\]
Also, across layer \(L_2\), we get \[P_{cond}=\frac{Ak_2(T_{12}-T_{23})}{L_2}=\frac{A0.900k_1(T_{12}-T_{23})}{0.700L_1}=\frac{A9k_1(T_{12}-T_{23})}{7L_1}=\frac{A9k_1(\Delta T_2)}{7L_1}~~~(2)\] Equating (1) and (2), we find \[\frac{144Ak_1(T_H-T_C)}{319L_1}=\frac{A9k_1(\Delta T_2)}{7L_1}\] \[\Delta T_2=\frac{(144)(7)(T_H-T_C)}{(9)(319)}=\frac{(144)(7)(30+15)}{(9)(319)}=15.80^\circ C\space(Answer)\] (b) \(k_2=1.1k_1\), we get \[P_{cond}=\frac{A(T_H-T_C)}{\frac{L_1}{k_1}+\frac{L_2}{k_2}+\frac{L_3}{k_3}}=\frac{A(T_H-T_C)}{\frac{L_1}{k_1}+\frac{0.700L_1}{1.1k_1}+\frac{0.350L_1}{0.800k_1}}=\frac{176Ak_1(T_H-T_C)}{365L_1}=\frac{Ak_1}{L_1}21.6986~~~(3)\] From (1), we got
\[P_{cond}=\frac{A(T_H-T_C)}{\frac{L_1}{k_1}+\frac{L_2}{k_2}+\frac{L_3}{k_3}}=\frac{A(T_H-T_C)}{\frac{L_1}{k_1}+\frac{0.700L_1}{0.900k_1}+\frac{0.350L_1}{0.800k_1}}=\frac{144Ak_1(T_H-T_C)}{319L_1}=20.3135~~~(1)\] Since, 21.6986 > 20.3135, the rate of heat transfer is more in (a) than (b).
- What would be the value of \(\Delta T_2\) across layer 2?
\[P_{cond}=\frac{Ak_2\Delta T_2}{L_2}=\frac{1.1k_1A\Delta T_2}{0.700L_1}~~~(4)\] \[\frac{11k_1A\Delta T_2}{7L_1}=\frac{Ak_1}{L_1}21.6986\] \[\Delta T_2=\frac{7}{11}21.6986=13.81^\circ C\space(Answer)\]
Problems:
Section 17-1 Temperature
[1] Suppose the temperature of a gas is 373.15 K when it is at the boiling point of water. What then is the limiting value of the ratio of the pressure of the gas at that boiling point to its pressure at the triple point of water? (Assume the volume of the gas is the same at both temperatures.)
[2] Two constant-volume gas thermometers are assembled, one with nitrogen and the other with hydrogen. Both contain enough gas so that \(p_3\) = 80 kPa. (a) What is the difference between the pressures in the two thermometers if both bulbs are in boiling water? (Hint: See Fig. 17-6.) (b) Which gas is at higher pressure?
Section 17-2 The Celsius and Fahrenheit Scales
[6] On a linear X temperature scale, water freezes at -125.0\(^\circ\)X and boils at 375.0\(^\circ\)X. On a linear Y temperature scale, water freezes at -70.00\(^\circ\)Y and boils at -30.00\(^\circ\)Y. A temperature of 50.00\(^\circ\)Y corresponds to what temperature on the X scale?
[7] Suppose that on a linear temperature scale X, water boils at -53.5\(^\circ\)X and freezes at -170\(^\circ\)X. What is a temperature of 340 K on the X scale? (Approximate water’s boiling point as 373 K.)
Section 17-3 Thermal Expansion
[8] At 20\(^\circ\)C, a brass cube has edge length 30 cm. What is the increase in the surface area when it is heated from 20\(^\circ\)C to 75\(^\circ\)C?
[11] What is the volume of a lead ball at 30.00\(^\circ\)C if the ball’s volume at 60.00\(^\circ\)C is 50.00 \(cm^3\)?
[19] A vertical glass tube of length L = 1.280 000 m is half filled with a liquid at 20.000 000\(^\circ\)C. How much will the height of the liquid column change when the tube and liquid are heated to 30.000 000\(^\circ\)C? Use coefficients \(\alpha_{glass} = 1.000 000 \times 10^{-5}\)/K and \(\beta_{liquid} = 4.000 000 \times 10^{-5}\)/K.
Section 17-4 Absorption of Heat
[23] A small electric immersion heater is used to heat 100 g of water for a cup of instant coffee. The heater is labeled “200 watts” (it converts electrical energy to thermal energy at this rate). Calculate the time required to bring all this water from 23.0 \(^\circ\)C to 100\(^\circ\)C, ignoring any heat losses.
[25] A certain diet doctor encourages people to diet by drinking ice water. His theory is that the body must burn off enough fat to raise the temperature of the water from 0.00\(^\circ\)C to the body temperature of 37.0\(^\circ\)C. How many liters of ice water would have to be consumed to burn off 454 g (about 1 lb) of fat, assuming that burning this much fat requires 3500 Cal be transferred to the ice water? Why is it not advisable to follow this diet? (One liter = \(10^3 ~cm^3\). The density of water is 1.00 \(g/cm^3\).)
Solution:
To burn 454 g = 454 \(\times 10^{-3} kg\) of fat requires Q = 3500 Cal = \(3500 \times 10^3~cal\) of heat. Note that food calorie is denoted by Cal, and heat calorie is denoted by cal. 1 Cal = 1000 cal.
Therefore, to burn 3500 Cal = \(3500\times 10^3\) cal, one has to drink ice water that can be calculated as follows:
\[Q = cm\Delta T\] \(m=\frac{Q}{c\Delta T}=\frac{3500\times 10^3~cal}{(1~Cal/g-K)(37^\circ C-0^\circ C)}=94.59\times 10^3 g\)
Volume is \(V = \frac{m}{\rho}=\frac{94.59\times 10^3 g}{1~g/cm^3}=94.59\times 10^3~cm^3=94.59~L\space(Answer)\)
“Drinking too much water can upset the balance of sodium in the blood, causing hyponatremia, which can be fatal.
- [26] What mass of butter, which has a usable energy content of 6.0 Cal/g (= 6000 cal/g), would be equivalent to the change in gravitational potential energy of a 73.0 kg man who ascends from sea level to the top of Mt. Everest, at elevation 8.84 km? Assume that the average g for the ascent is 9.80 \(m/s^2\).
Solution:
The mass of butter requires to climb from the sea level to the top of Mt. Everest at an elevation of 8.84 km can be calculated as
\[m_{man}gh=m_{butter}(6000\space cal/g)(4.1868\frac{J}{cal})\]
where 1 cal = 3.968 \(\times 10^{-3}\) Btu = 4.1868 J.
\[m_{butter}=\frac{m_{man}gh}{(6000\space cal/g)(4.1868\frac{J}{cal})}\] \[m_{butter}=\frac{(73.0~kg)(9.8~m/s^2)(8.84~km)}{(6000\space cal/g)(4.1868\frac{J}{cal})}=0.25175~kg=251.75~g\space (Answer)\] 11. [41] (a) Two 50 g ice cubes are dropped into 200 g of water in a thermally insulated container. If the water is initially at 25 \(^\circ\)C, and the ice comes directly from a freezer at -15\(^\circ\) C, what is the final temperature at thermal equilibrium? (b) What is the final temperature if only one ice cube is used?
Section 17-5 The First Law of Thermodynamics
- [44] A thermodynamic system is taken from state A to state B to state C, and then back to A, as shown in the p-V diagram of Fig. 17-38a. The vertical scale is set by \(p_s\) = 40 Pa, and the horizontal scale is set by \(V_s\) = 4.0 \(m^3\). (a)–(g) Complete the table in Fig. 17-38b by inserting a plus sign, a minus sign, or a zero in each indicated cell. (h) What is the net work done by the system as it moves once through the cycle ABCA?
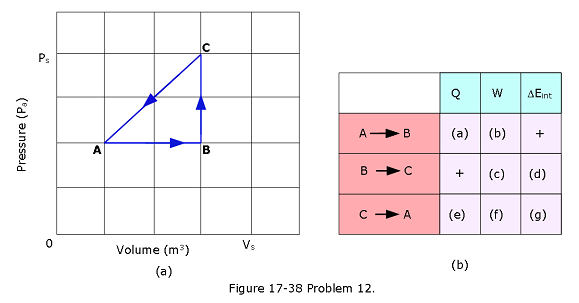
- [45] A gas within a closed chamber undergoes the cycle shown in the p-V diagram of Fig. 17-39. The horizontal scale is set by \(V_s\) = 4.0 \(m^3\). Calculate the net energy added to the system as heat during one complete cycle.
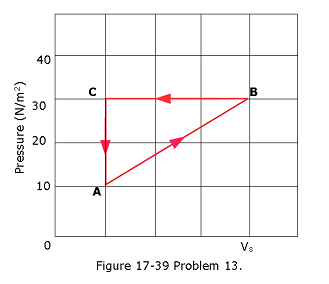
- [50] A lab sample of gas is taken through cycle abca shown in the p-V diagram of Fig. 17-43. The net work done is +1.2 J. Along path ab, the change in the internal energy is +3.0 J and the magnitude of the work done is 5.0 J. Along path ca, the energy transferred to the gas as heat is +2.5 J. How much energy is transferred as heat along (a) path ab and (b) path bc?
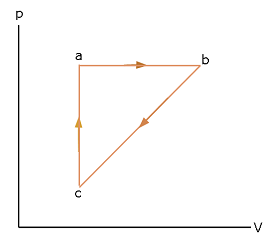
Section 17-6 Heat Transfer Mechanisms
- [52] The ceiling of a single-family dwelling in a cold climate should have an R-value of 30. To give such insulation, how thick would a layer of (a) polyurethane foam and (b) silver have to be?
[Note:Thermal Resistance to Conduction (R-Value) is defined as \(R=\frac{L}{k}\). The lower the thermal conductivity, k, of the material of which a slab is made, the higher the R-value of the slab; so something that has a high R-value is a poor thermal conductor and thus a good thermal insulator.]
Solution:
- Given the R-value, \(R= 30 \frac{ft^2.^\circ F.h}{BTU}\)
Additional Problems